Research Directions
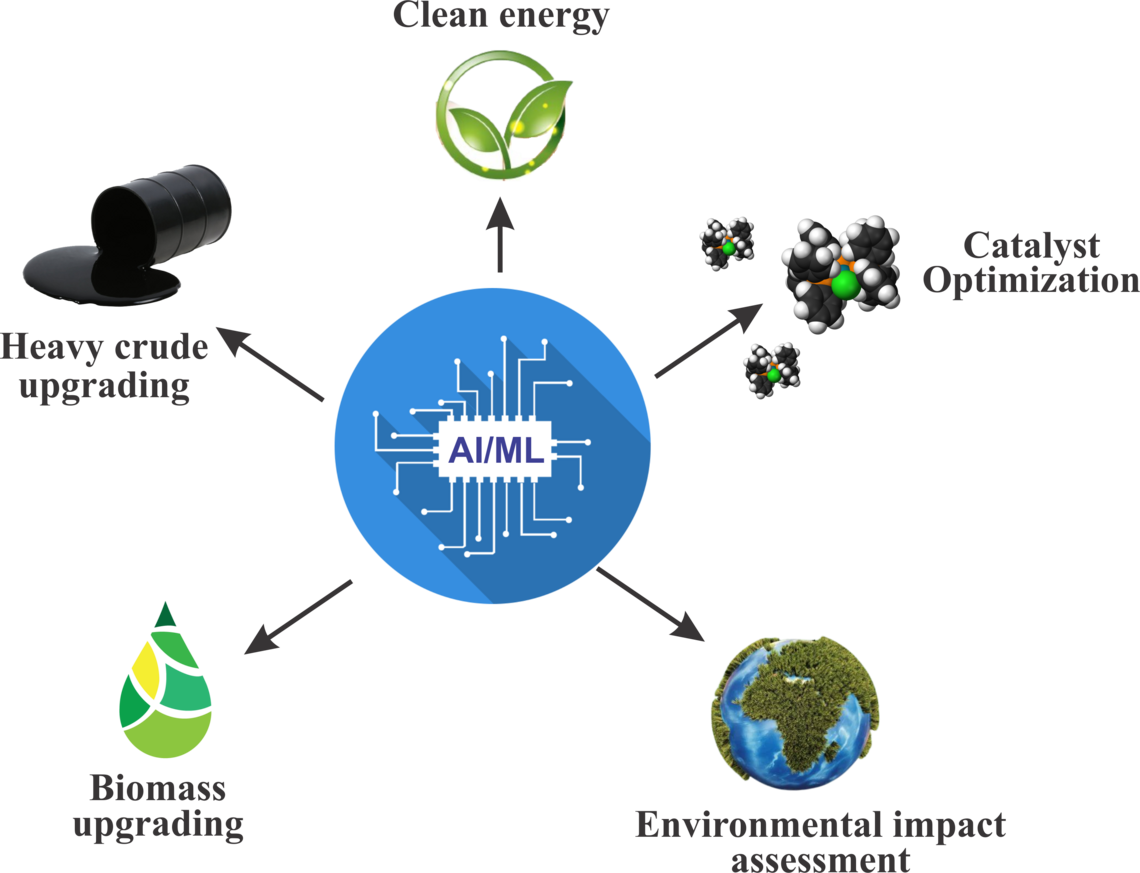
Artificial intelligence/machine learning for clean energy conversion
The integration of AI/ML techniques in catalytic upgrading and clean energy conversion processes offers transformative opportunities across multiple sectors. AI/ML-driven catalyst design optimization accelerates the discovery of efficient catalysts for heavy crude oil, light olefin, and biomass upgrading, enabling tailored solutions for diverse feedstocks. Process optimization, facilitated by real-time monitoring and decision-making, enhances productivity, reduces energy consumption, and improves economic viability, leading to sustainable and cost-effective upgrading solutions. AI/ML methodologies also play a crucial role in the development of low-cost sorbents for natural gas storage, ensuring safer and more accessible utilization in vehicular applications. Additionally, AI/ML-driven waste conversion processes contribute to sustainable waste management practices by converting municipal solid waste and greenhouse gases into valuable commodities such as liquid fuels or chemicals. Furthermore, AI/ML-driven optimization of low-temperature catalysts for NOx control under high sulfur environments, addressing environmental challenges in emissions reduction. Finally, AI/ML-driven optimization of non-thermal plasma and photocatalytic conversion processes advances energy efficiency and reduces environmental impact, paving the way for cleaner and more sustainable fuel production. Collectively, AI/ML-driven advancements drive innovation, efficiency, and sustainability in the energy sector, supporting the transition towards a greener and more resilient energy landscape.

Photoelectrochemical Methane Activation and Utilization
Methane holds promise as a cleaner energy source, but it presents a major challenge as a potent greenhouse gas, being at least 25 times the warming potential of carbon dioxide (CO2) over a 100-year period. Methane is estimated to be responsible for 30% of observed global warming to date and the level of atmospheric methane continues to rise. To address this issue, scientists are focusing on liquefying methane for easier handling and transport, and converting methane into valuable chemicals such as methanol, ethanol, propanol, and ammonia. This transformation not only contributes to sustainable energy development but also helps reduce carbon emissions, offering a path to a greener future.
While several methods, including steam reforming, electro-catalysis, and plasma-catalysis, have been explored in the quest to transform methane into valuable chemicals like methanol, ethanol, formic acid, and propanol, none of these approaches have successfully scaled up to efficient industrial levels. This presents a unique opportunity for cutting-edge research to bridge the gap and enable effective methane utilization. One particularly promising avenue is the Photo-electro-chemical (PEC) technique, which combines photochemical and electrochemical processes, offering efficient charge separation with the help of external fields. The potential of the PEC technique making its way to the marketplace is significant if scientists can devise efficient and cost-effective methods for using solar light to convert methane into higher-value chemicals. However, PEC technology has received limited attention, and the key challenge lies in achieving mass production while ensuring substantial solar light absorption, high reaction rates, and selectivity. In this context, our research emerges as a beacon of progress, with the goal of providing a comprehensive solution to these persistent challenges and contributing to climate change mitigation by converting methane into high-value products at near-ambient conditions.
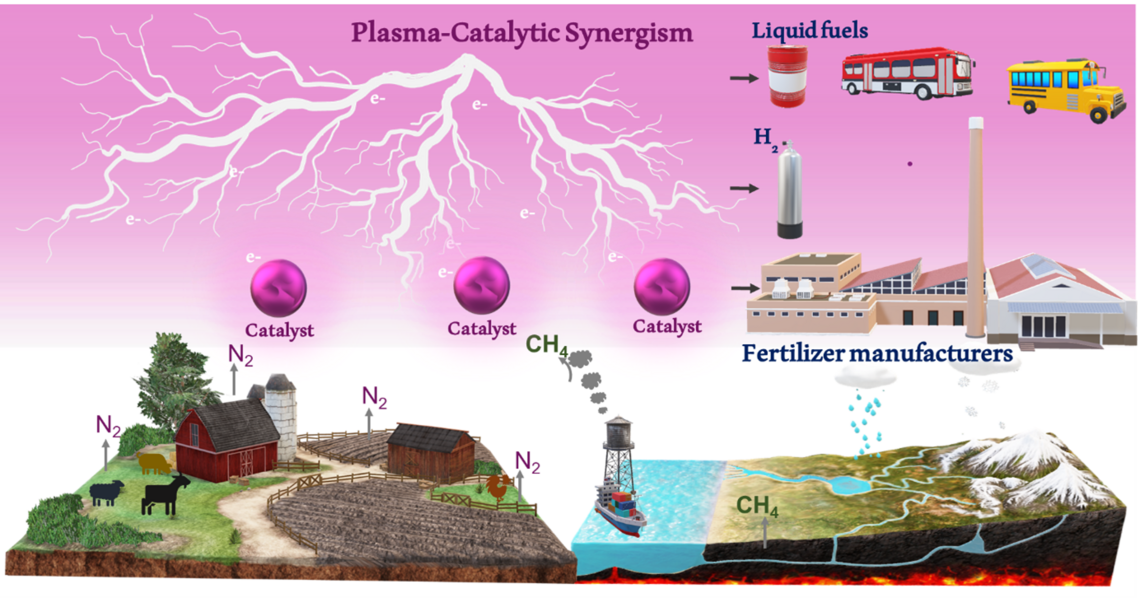
Sustainable Plasma Catalytic Ammonia Production from Methane and Nitrogen
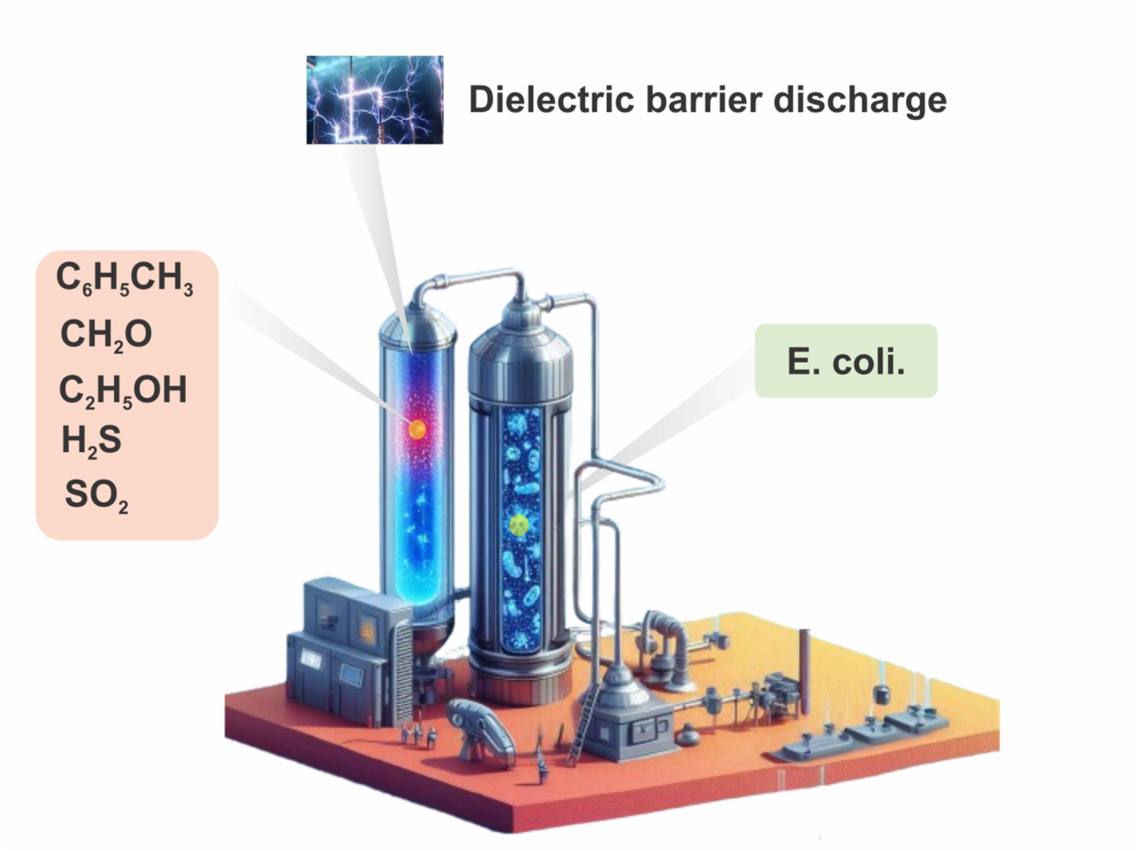
Nonthermal Plasma Assisted Air Purification and Sterilization
Air pollution poses a significant threat to environmental and human health, necessitating the exploration of innovative technologies for effective pollution control. Non-thermal plasma (NTP) has emerged as a promising solution due to its dynamic nature and versatile applications. During NTP, high-energy state of matter is created by applying electrical energy to a gas, which predominantly transfers energy to electrons rather than increasing the temperature of the gas itself. In NTP, electrons become highly energetic, leading to a state where their temperature is significantly higher than that of the surrounding gas molecules. This unique condition promotes various chemical reactions and processes that typically do not occur at ordinary temperatures, including electron dissociation processes and molecular excitation. NTP utilizes ionized gases to neutralize pollutants, providing a more effective and sustainable alternative to traditional methods. By harnessing the power of atmospheric plasma, the harmful effects of industrial activities on air quality can be mitigated, offering a ray of hope for a cleaner and healthier environment. This research aims to develop a NTP assisted air purification and sterilization process for air quality improvement and bacterial disinfection.
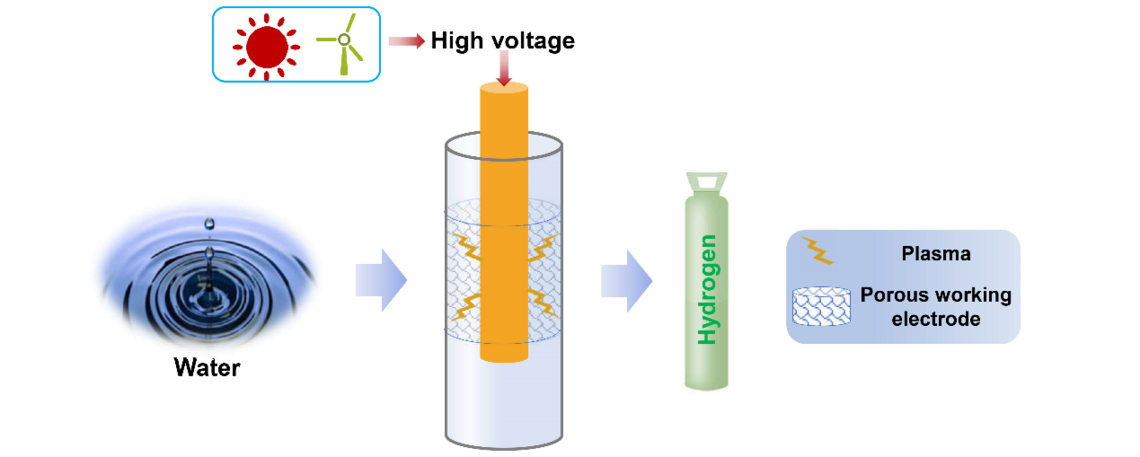
Nonthermal Plasma Assisted Hydrogen Production via Water Splitting

Catalytic Heavy Crude Oil Upgrading Using Natural Gas
Hydrocracking is conventionally employed as the process to upgrade heavy crude oil for reducing its viscosity, increasing its H/C ratio, and removing associated impurities. Such process is costly and energy inefficient due to the involvements of expensive hydrogen generated from a separate unit through natural gas steam reforming and high operation pressure. The proposed research direction aims to effectively upgrade heavy crude oil including extra crude oil and bitumen extracted from oil sands to synthetic crude oil with acceptable specifications (i.e. API of 30 ~ 34o and sulfur content of 0.1 ~ 0.2%) and increased productivity at much lower pressure (<3 MPa) through directly using natural gas as reducing agent. Under the facilitation of the specially developed catalyst, methane as the main component of natural gas can be activated and thereafter crack down and saturate the long carbon chain and remove heteroatoms contained in the heavy crude oil, while itself being converted to produce extra synthetic crude oil. The successful achievement of the promised objectives will lead to significant cost reduction of the heavy crude oil upgrading process and make it more economically attractive. Moreover, the outcomes obtained from the proposed research will create a novel way of methane activation and liquefaction, leading to the breakthrough in the field of catalysis and its application in clean energy conversion.
Catalytic Light Olefin Upgrading - Using Natural Gas for Gasoline Quality Improvement
Traditionally, thermal cracking or visbreaking is widely employed to break down the long carbon chain of the high molecular weight residues for significantly lowering down its viscosity. Usually, such treatment will result in a relatively higher content of unsaturated light olefins in the formed synthetic oil which will create detrimental effects on its thermal stability during long-term storage and generate negative environmental impact when combusted. Olefins contained in the produced oil are oxidatively and thermally unstable and may gradually form polymeric deposits during storage. Such carbonaceous gum formation on the fuel injector and in the engine’s intake system will lead to gasoline insufficient combustion, resulting in increased hazardous emissions such as CO, hydrocarbon, and NOx. Moreover, these olefinic hydrocarbons itself might also contribute to photochemical reactions in the atmosphere, resulting in the formation of photochemical smog in susceptible urban areas. In addition, the direct release of olefins to the atmosphere might be closely related to ozone formation and toxic dienes. Therefore, hydrotreating is widely employed as the established industrial practice to saturate olefins for gasoline quality improvement. However, the involvement of such an expensive hydrogen resource which is not naturally available results in the significant cost increase of this upgrading step. It is thus critical to find out an alternative substitute that is readily available and abundant in nature for prominent upgrading cost reduction, making the whole process more economically attractive.
Under such background, developing a technology that can effectively convert natural gas into valuable chemicals will definitely attract the attentions from both industry and academia. The proposed project aims to develop a catalyst system and associated process which can efficiently convert natural gas into extra oil during the cracked distillates upgrading process. At the same time, the light olefins present in cracked oil will be sufficiently saturated into paraffin for improving the quality of the produced oil under the facilitation of the introduced natural gas.
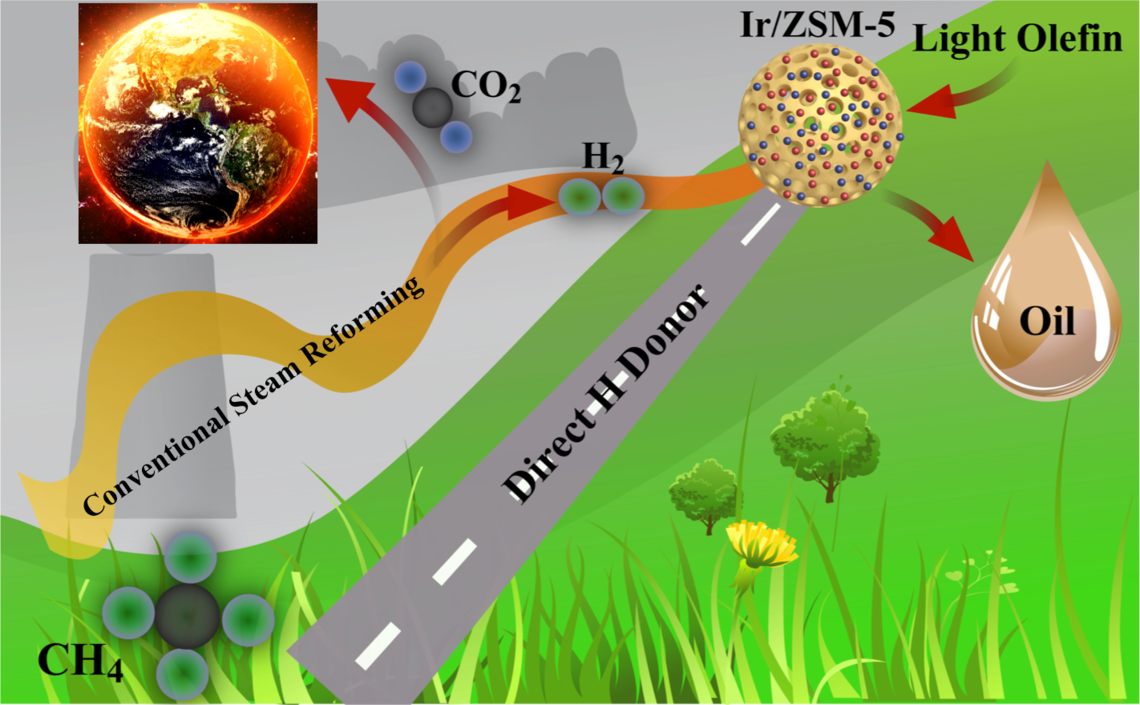
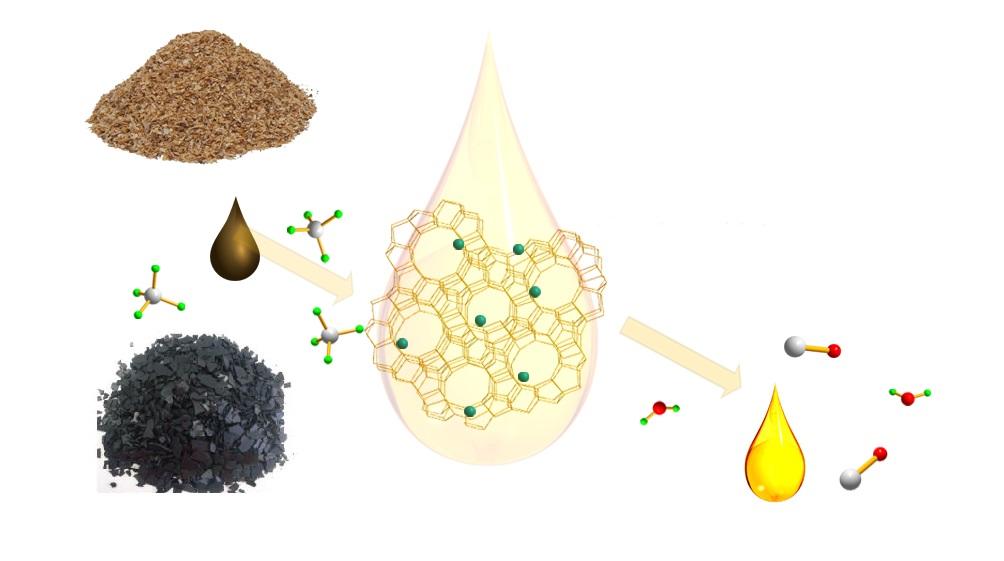
Catalytic Coal or Biomass Upgrading Using Natural Gas for Liquid Chemical or Fuel Productions
Biomass or low-rank coal fast pyrolysis followed by hydrodeoxygenation upgrading is the most popular way to produce synthetic oil. Such a two-step process can be combined together as so-called hydropyrolysis treatment. This approach usually involves continuous hydrogen flow, resulting in significantly increased operating costs. Compared to hydrogen which is not naturally available and mainly produced through methane steam reforming, methane can be readily obtained from nature known as natural gas with a low cost. The proposed research is planning to directly employ methane as a reducing agent instead of hydrogen for removing oxygen from biomass under the facilitation of specially developed low-cost supported catalyst, leading to significantly reduced operation cost. Moreover, methane itself will be converted to higher hydrocarbons beneficial for extra liquid fuel production. In addition, the char generated from the aforementioned process is conventionally discharged out and delivered to a separate process for syngas production or power generation from combustion. Unlike such a separate char processing route, we propose to use a single step to simultaneously convert char and volatile matters from biomass pyrolysis to syngas and liquid fuel, during which char is gasified by water vapor or CO2 generated from methane deoxygenation of volatile matters under the facilitation of co-added gasification catalyst. Later on, such a gasification catalyst will be engineered together with tar upgrading catalyst to serve as one catalyst with multifunction. A novel circulating bed reactor design composed of a transport reactor equipped with recycle leg and two consecutive gas cyclones grant the proposed catalytic process with the feature of continuous biomass solid feeding and ash removal while allowing catalyst and unreacted solid biomass recycled back for further reaction, leading to minimization of catalyst make-up and extended residence time for better solid fuel conversion and thus benefiting its potential commercialization.
Natural Gas Solid Sorbent Development for Vehicular Application
Although there are over 13 million natural gas vehicles on the road worldwide due to its less corrosion to engine and emissions to the environment compared to a gasoline-fueled car, all of them are fueled with compressed natural gas (CNG) with pressure up to 250 bar in order to compensate the disadvantage brought by the low volumetric energy density of natural gas. Running a car filled with such high-pressure natural gas is very dangerous. Therefore, in order to take advantage of the low-cost feature of natural gas due to its massive reserves including the recent big discovery of shale gas, there is an immediate need for low-cost solid sorbent for natural gas storage at a much lower pressure (<35 bar), beneficial for direct home refueling and much safer driving. Unlike the cost-prohibitive organic containing solid sorbent intensively studied currently, after appropriate tailoring (e.g. surface decorating and pore engineering), our carbon-based solid sorbent can have significantly lower cost with comparable or even better performance on natural gas adsorption/desorption, making it very attractive for transportation application at large scale.

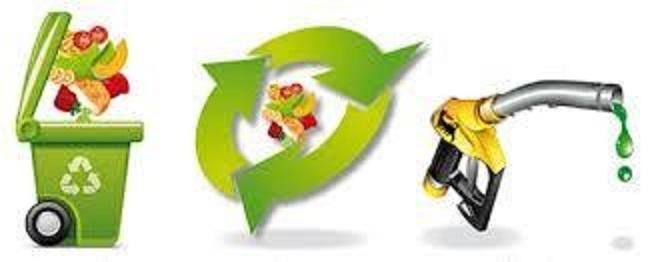
Catalytic Conversion of Municipal Solid Waste and Greenhouse Gas into Valuable Commodities
Along with human’s urbanization and industrialization, more and more municipal wastes have been generated, which create significant environmental hazards and high processing cost during its storage and disposal. Compared to simple burying or combustion which is widely employed currently for solid waste disposal, sorting and recycling recyclable materials might be a better way to lessen the environmental burden derived from waste disposal and reuse their remaining value. However, such activity is lack of policy support and economic stimulus, resulting in people’s reluctance to do so. On the contrary, if municipal waste can be effectively converted to value commodities such as liquid fuel with minimal environmental impact, it will become a completely different story.
Carbon dioxide is another environmental pollutant typically generated from fossil fuel combustion, causing global warming. In order to keep using fossil fuel to power our world without sacrificing too much of our future, CO2 capture and sequestration have been vigorously studied in recent years. Compared to simply injecting captured CO2 into the ground for storage, CO2 reuse to make valuable products such as liquid fuel with minimized external energy input sounds more economically attractive.
Our study aims to develop a novel process where municipal waste and CO2 will be simultaneously converted to valuable liquid chemicals or fuels. During this process, nanotechnology will be applied to invent a specially tailored catalyst which can effectively catalyze the CO2 gasification of municipal waste under moderate temperature (600 ~ 800 oC). The produced CO-rich gas will undergo a water-gas shift (WGS) reaction to achieve a desirable CO to H2 ratio immediately followed by a liquefaction process under the facilitation of another developed novel catalyst system at a temperature of 350 ~ 450 oC. The unreacted CO2 will be recycled back to the gasifier for further reaction. The whole process will be operated under near atmospheric pressure, effectively avoiding the pressure mismatch between gasification and conventional Fischer-Tropsch (FT) process. Such operation will lead to significant capital and operation cost reduction due to the elimination of the expensive pressurization step before the liquefaction process and usage of less costly fabrication materials as well as better liquefier design for efficient reaction heat dissipation to prevent catalyst deactivation.
Catalytic Natural Gas Conversion to Liquid Chemicals or Fuels
In addition to the aforementioned nonoxidative methane activation, the methane activation has also been extensively studied under the oxidative environment through the Oxidative Coupling of Methane (OCM) reaction to produce ethylene, a very important petrochemical feedstock, since 1980s. Although hundreds of catalysts have been tested since then, their poor C2 product yields (<25%) limit the further commercial considerations of such a process. The conventional cryogenic separation of ethylene from methane is very energy-intensive and not economically feasible for a stream with such low ethylene concentration. Under such circumstance, rather than keeping trying to optimize catalyst formulation for slightly enhanced performance which might be internally limited by the reaction thermodynamics, we propose to couple this OCM reaction with the following oligomerization reaction in sequence to make liquid chemicals or fuels as the final products.
A mixture of ethylene, ethane, and methane will be first obtained from an oxidative coupling of methane (OCM) reaction through chemical looping operation using a state-of-art OCM catalyst and then sent to a separate reactor for liquid formation reaction under the facilitation of an exclusively designed catalyst, through which most of the C2 species (>90%) and part of the methane (>20%) will be transformed to liquid. The remaining unreacted methane and other residual gas products will be recycled back to the first OCM operation for further reaction. As a result, very high natural gas to the liquid conversion will be achieved through this novel two-step approach. Moreover, even higher conversion and operation efficiency will be expected when shale gas, the special form of natural gas, is used as the feedstock due to its relatively higher contents of C2 (~10%) and C3 (~5%) components.
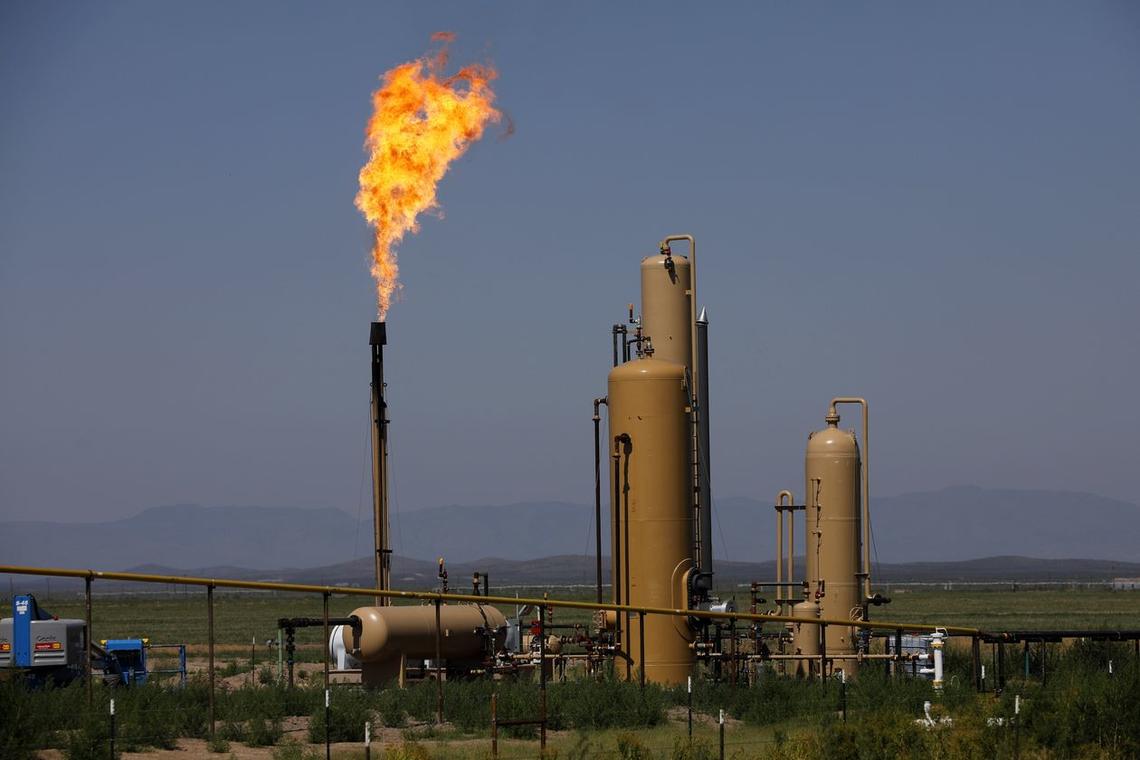
Image source: www.wsj.com/articles/the-natural-gas-market-is-in-a-summer-meltdown-11564163729
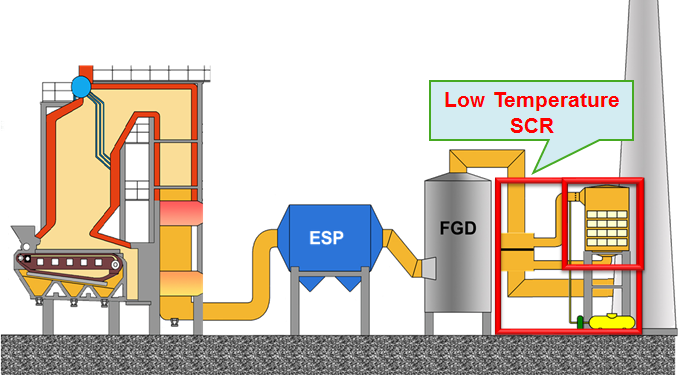
Low Temperature Catalytic NOx Control under High Sulfur Environment
NOx is a well known hazardous gas which can not only form acid rain and destroy ozone, but also cause respiratory diseases of human body. There are multiple methods developed currently to remove NOx. Among them, selective Catalytic Reduction, SCR, represents the most widely used and efficient post-combustion technique for mitigation of NOx emissions from stationary combustion sources. The most common SCR process for coal-fired power plants is the high-dust (HD) configuration, in which the SCR catalyst is located between downstream of economizer and upstream of the precipitator or other particle collection devices and processes the full dust loading leaving the boiler. The reason for HD configuration is because of the desired reaction temperature of 500-700 °F at this configuration. However, HD configuration can cause significant catalyst deactivations by mechanism of masking, fouling, and poisoning. If a catalyst that could efficiently remove NOx at a relatively lower temperature 350 °F, the typical temperature downstream of particle devices, and can tolerate high concentration SO2 as well as H2O, then fast deactivation problems at HD configuration could be easily avoided. However, such a low temperature SCR catalyst development still remains a big challenge nowadays faced by the entire catalysis field.
Although the amount of flying ash from coal combustion is significantly reduced after the electrostatic precipitator (ESP), the low temperature makes a notably negative impact on the achievement of similar catalytic activity as that obtained at hot side. This type of catalyst deactivation is physical and catalyst can therefore be regenerated easily by simply washing or heating up to slightly higher temperature. Furthermore, the formation of ammonium sulfate or sulfite on the catalyst surface has a higher tendency at low temperature end than at hot end, which will cover the active sites of the SCR catalyst, resulting in the catalyst deactivation. In addition, the presence of high concentration water vapor will create more unfavorable influence on the maintenance of high activity at cold side than at hot side due to pore condensation, although its influence is reversible. If a chemical bond is formed between the active metal containing in the catalyst and adsorbed SOx, the formed metal sulfite or sulfate will permanently poison the catalyst and is very difficult to be removed from the catalyst surface even under high temperature.
Based on aforementioned challenges to do NOx removal in flue gas from high sulfur coal-fired power plant at low temperature, the conventional high temperature SCR catalyst is not suitable to address all the difficulties facing at cold side. It is critical to develop a new catalyst which can remain active under such harsh environment.
Catalytic Paraffin-rich Oil Upgrading under Methane
Aromatic compounds have been considered as important intermediates in the production of chemical commodity products. Among them, the so-called BTX family, referred to benzene, toluene and xylenes, are of great interest in petrochemical industry due to their application on the formation of high value-added chemicals as the fundamental building blocks. Catalytic aromatization of paraffin-rich oil like naphtha and raffinate oil which are abundant in crude oil and reaction intermediates in petrochemical refineries has been considered as an effective pathway for BTX aromatic production. Unlike the typical approach where inert or H2 atmosphere is engaged, this study has systematically investigated the effect of methane on the aromatization of paraffin-rich oils and found out the presence of methane can effectively promote the formation of monoaromatics, particularly BTX. In other words, BTX selectivity is significantly enhanced when methane is engaged as gas environment.
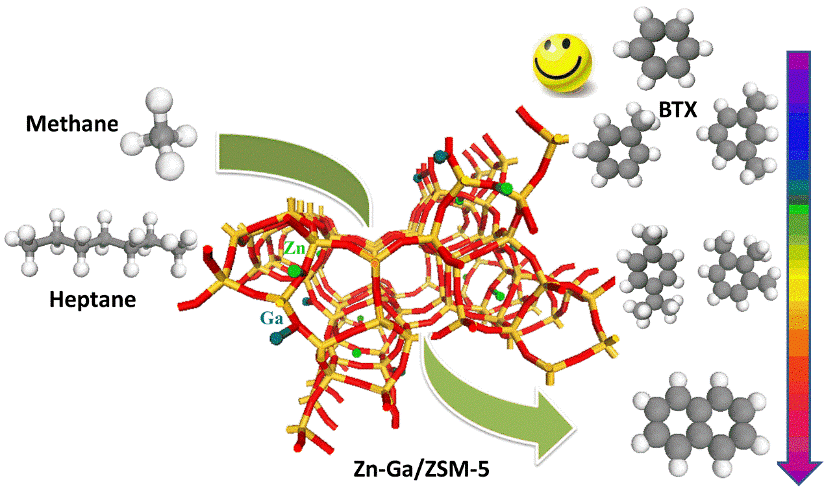
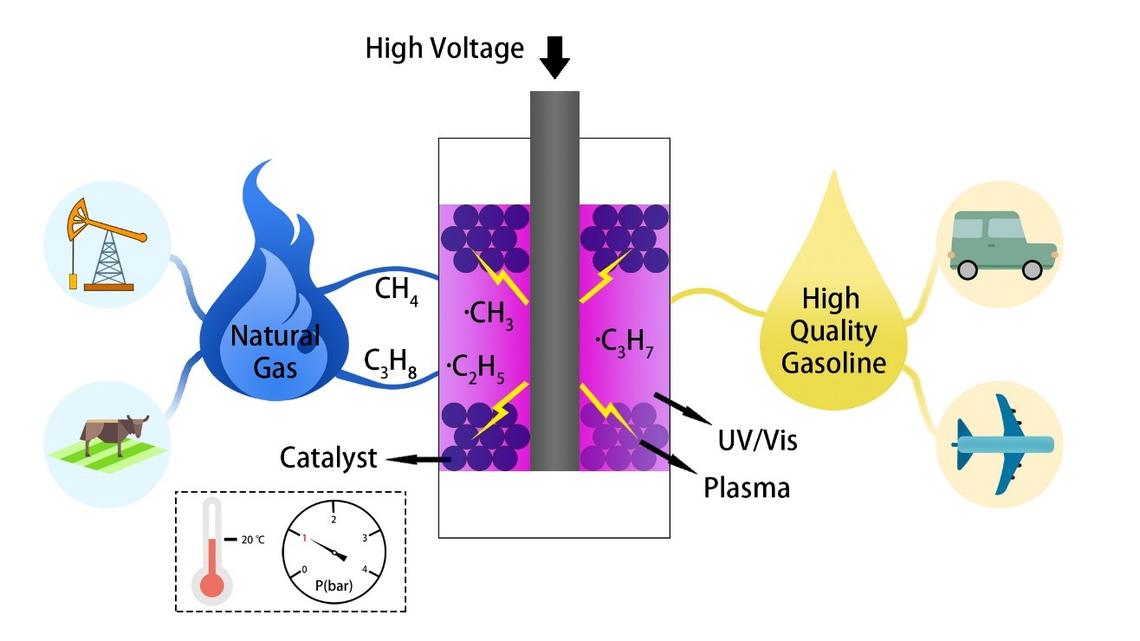
Non-thermal Plasma Assisted Photocatalytic Conversion of Light Hydrocarbons to Liquid Fuels
Low-cost light hydrocarbons like natural gas, biogas, and liquefied petroleum gas are abundant, cheap and underutilized resources with increasing proved reserves as well as an agricultural byproduct. Currently, they mainly used as sources of energy for heating, cooking, and power generation. Increasing worldwide energy demands with the discovery of huge shale deposits has stimulated research interests in the conversion of these light hydrocarbons to high-value transportation fuel or petrochemicals. Our research group pioneers an innovative non-thermal plasma assisted photocatalytic approach to convert these low-cost light hydrocarbons to high value-added liquid fuels/chemicals at ambient conditions with significantly enhanced energy efficiency and reduced coke formation. Our proof-of-concept investigations highlight the synergy of plasma activation and photocatalysis in the upgrading of light hydrocarbons. Higher than 50% light hydrocarbons conversion and more than 60% liquid yield abundant with branched C6-C9 paraffins with limited coke formation (<5%) are experimentally witnessed at ambient conditions, which opens a brand new door for light hydrocarbons valorization and thus greatly reduces the heavy dependence on finite other fossil fuels such as oil and coal.