Large-scale Parallel Computing of Vibrational Spectra in the Framework of Auxiliary Density Functional Theory
Vibrational spectroscopy is a valuable tool in modern chemistry for molecular structure elucidation, reaction monitoring and quality control. The two most common variants are the infrared (IR) and Raman spectroscopy. These non-destructive techniques can measure vibrational energy levels associated with the chemical structure in a sample. and provide insight into the nature of chemical bonds and intramolecular forces acting between the atoms in a molecule. To extract such information it is necessary to assign vibrational motions to each peak in a spectrum. However even for experienced chemists, a vibrational mode assignment can be a rather challenging task. In the framework of Kohn-Sham-DFT, the simulation of vibrational spectra is proportional to the second-order energy-derivatives with respect to all possible nuclear displacements.
Such calculation involves the solution of the coupled-perturbed Kohn-Sham (CPKS) equations. Although iterative solvers are employed due to the large dimensionality of the corresponding CPKS equation systems, vibrational spectra calculations are still formidable tasks because a CPKS equation system has to be solved for each nuclear displacement, even for systems with less than hundred atoms.
An alternative approach to CPKS was developed in the framework of auxiliary density functional theory (ADFT) , where the calculation of the four-center electron repulsion integrals is avoided by introducing an auxiliary function basis for the variational fitting of the Coulomb potential, can provide a reduction in dimensionality and a direct solution of the perturbation equation system, analog to the CPKS equations. This so-called auxiliary density perturbation theory (ADPT), has been already successfully used to calculate static and dynamic polarizabilities, as well as spin-spin coupling constants and Fukui functions. This perturbation theory can be extended for the calculation of second-order electronic energy derivatives with respect to nuclear displacements. As a result, an efficient route for the calculation of IR and Raman spectra in the framework of ADFT becomes available through ADPT, currently implemented in the density functional theory program deMon2k.
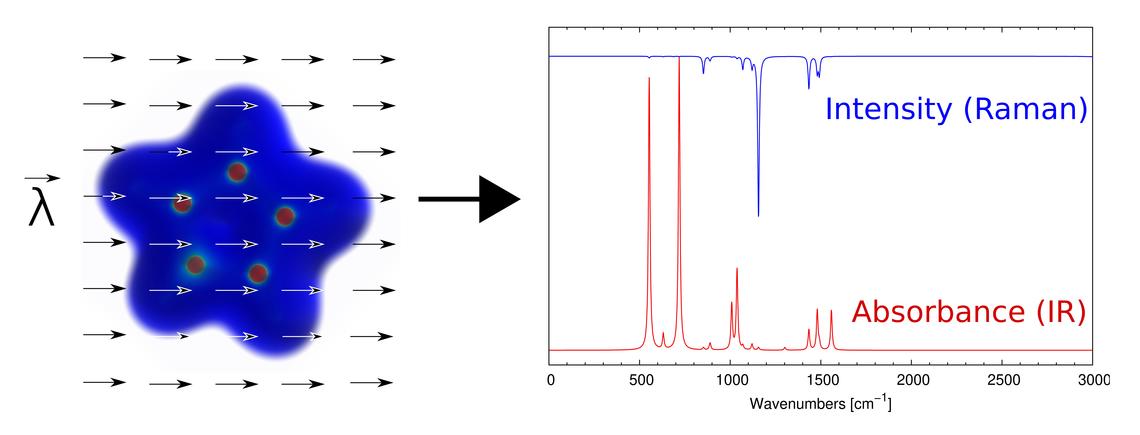
Figure: The electron density response due to nuclear displacements and electric fields allows to simulate IR and Raman spectra.
Theoretical Modelling of Partial Heavy Oil Upgrading
Along with its many other industrially interesting properties, molybdenum disulfide is being developed in the Pereira group as a hydrogenation and coke-prevention catalyst for in situ heavy oil upgrading. In the computational part of the project, we have used DFT with deMon2k to explore the interactions between and among a MoS2 nanoparticle, an asphaltenes model and models for calcium carbonate and silica. Furthermore, deep Behler-Parrinello neural network model is being developed as an alternative approach for studies of larger MoS2 nanoparticles. This work has a potential to vastly increase the applicability range of the methodology especially when used within an adaptive Born Oppenheimer molecular dynamics approach.
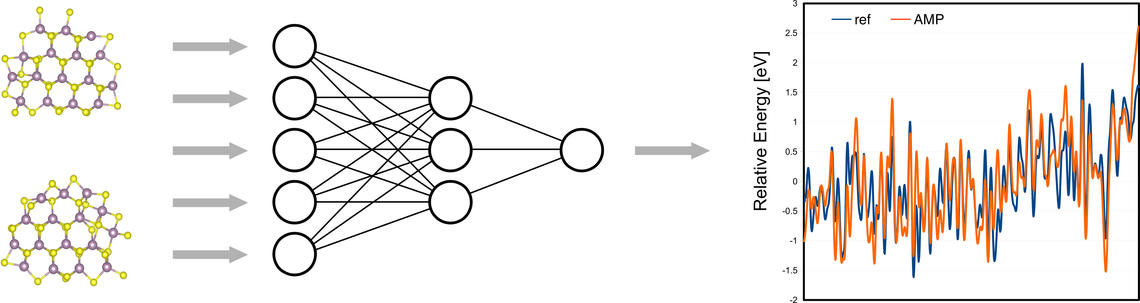
Figure: Diagram showing development of deep neural network model for molybdenum disulfide.
Hydrorefined Fuels - Computational Insight Into the Mechanism
With collaboration with a Calgary energy startup company, we have been working on the computational modelling of upgrading heavy fuels and fuel emulsions in order to study clean fuels with less greenhouse emissions. Classical and quantum mechanical models are used in order to study the stability and composition of fuel products.
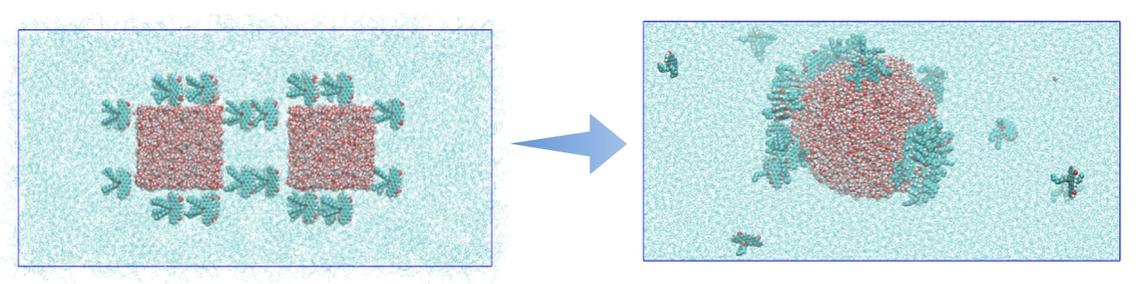
Computational Investigation of Ni-Ceria Nanocatalysts to Produce Hydrogen in Aquaprocessing of Heavy Oil
The use of unconventional heavy oil, such as the bitumen derived from oil sands, has progressively increased, placing these materials as a compelling alternative to meet short- and long-term energy demands. Nevertheless, the current technologies available to exploit and upgrade this crude have critical challenges, such as high production costs, lower energy efficiency, and, even worse, significant environmental damage. Recently, nanocatalysts have emerged as a feasible technology for the in-situ upgrading of heavy oil. In this procedure, a hot fluid that carries the metallic nanoparticles is injected into the reservoir, where the particles act as a catalyst, accelerating hydrocarbon hydrogenation and cracking. Nevertheless, due to the complexity of the process, involving many chemical reactions that occur deep in the reservoir, significant experimental and theoretical studies are still necessary to fully understand this technique and use it at the industrial level. In this project, we are performing quantum chemistry calculations to investigate the role of NPs, specifically Ni-Ceria, in the in-situ upgrading technology and the aquaprocessing of oil sands.
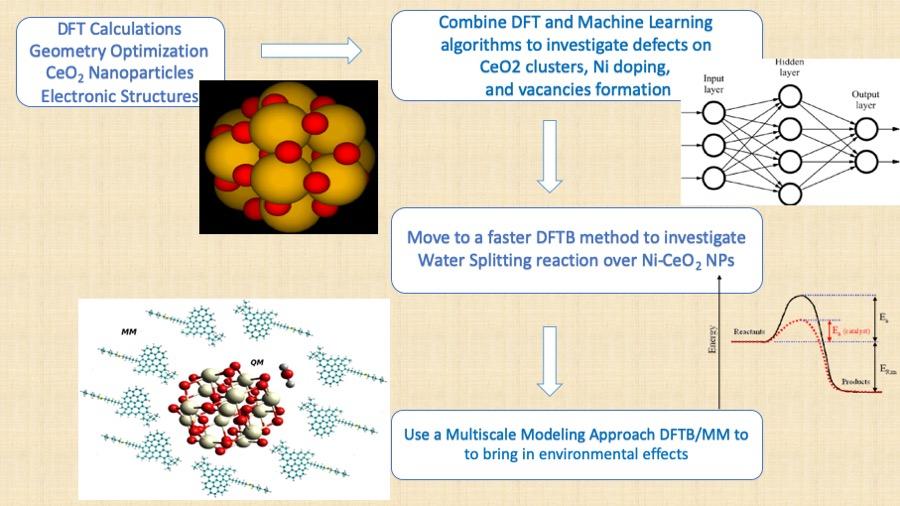
Parameterization of classical Drude polarizable force field
Classical force-field models for molecular dynamics (MD) simulations, such as that depicted in Fig. A, require extensive parametrization in order to be consistent with both quantum predictions, as well as experimental data on macroscopic properties (such as solvation free energy). However, explicit account of polarizability is critical for descriptions of regions of high polarizability, such as binding sites for ions and/or charged ligands. The Drude polarizable model (Fig.B) is one such model which adds a virtual Drude particle to all atoms of the CHARMM36 force-field to account for polarizability to a dipole approximation. My current research focuses on ways to fit this model in order to reproduce both QM and thermodynamic properties. Fig.C depicts the results of lowering existing Lennard Jones parameter for potassium ion and acetate interaction, which result in a better agreement between the classical Drude and QM interaction energy of the gas-phase interaction. Validation of the new parameter set is performed using osmotic pressure calculation (Fig.D) from 10ns MD simulations of an acetate-potassium aqueous salt solution constrained by a semi-permeable membrane.
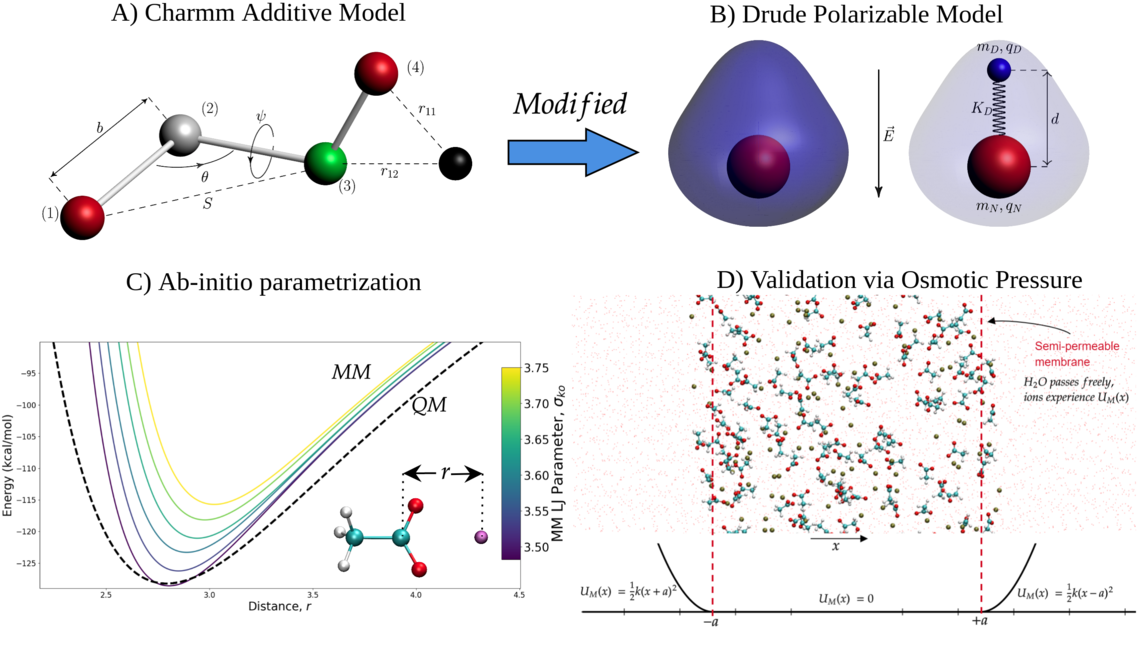
QM/MM Study of Ion Transporter Proteins
Analyzing energetic of ion transport across protein channels is crucial for understanding their biological function. Accurate estimate of energy in ion transport requires a good representation of atoms and adequate sampling. In this project we employ QM/MM representation of the ion transporter wherein the putative binding sites of the protein, ion and nearest water are modeled using a QM representation, while the rest of the system is modeled using an MM (MD) representation. We will also explore new algorithms to improve the sampling of the energy.
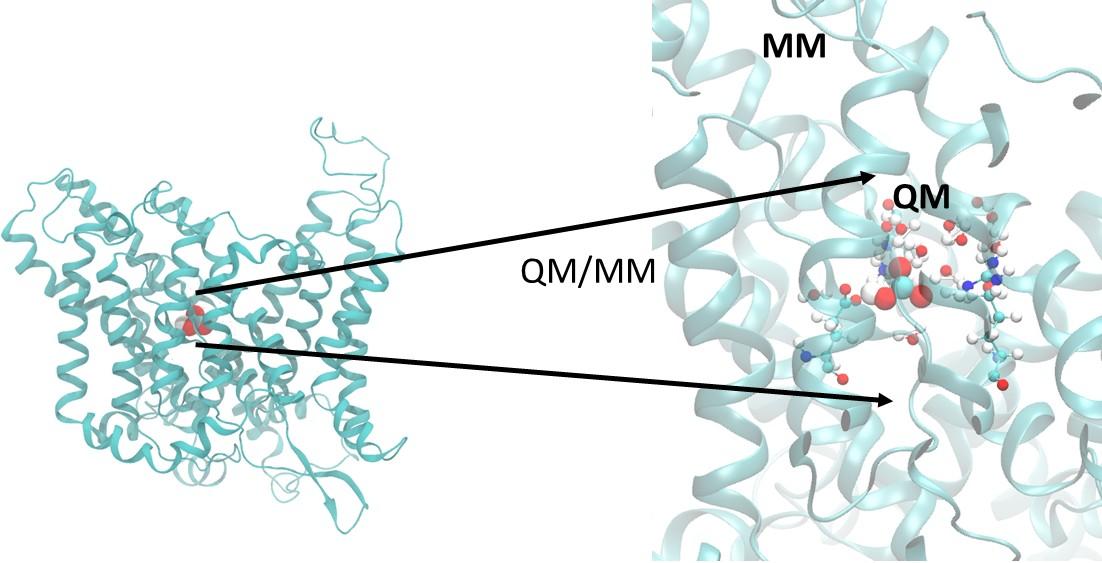